Introduction
The global demand for chocolate is increasing, and its appeal is growing due to its physiological and health-enhancing effects (Katz et al., 2011; Montagna et al., 2019). These health benefits of chocolate are mainly attributed to the antioxidant properties of flavonoids found in cocoa (Faccinetto-Beltran et al., 2021). Molten chocolate is a suspension of particles of sugar, cocoa solids, and/or milk powder in which cocoa butter, mixed with other fats, serves as the continuous phase (Rhom et al., 2018; Vasquez et al., 2019). This concentrated suspension is a crucial ingredient in the food processing industry for the production of various products, including ice cream and bakery items. The flow characteristics of this suspension play a significant role in subsequent processing stages, as they greatly influence the final texture and appearance.
The rheological properties of these products are influenced by a number of processing techniques, including refining, conching, and tempering. These properties are dependent on several process parameters, including time and temperature, particle size distribution, and the amount of fat present in the product (Afoakwa et al., 2009; Faccinetto-Beltran et al., 2021). Previous studies have demonstrated that the apparent viscosity of dark chocolate increases with higher sugar content and lower fat solids content (Fernandes et al., 2013; Vasquez et al., 2019). Furthermore, it has been observed that molten chocolate displays a shear-thinning behavior, characterized by a yield stress and a plastic viscosity (Fernandes et al., 2013; Rohm et al., 2018; Quispe-Chambilla et al., 2022). It was also demonstrated that the rheological properties were affected by the intensity of grinding and could be targeted (Rohm et al., 2018). Quispe-Chambilla et al. (2022) found that the partial addition of food ingredients, such as peanut paste and Sacha Inchi, significantly impacted the rheological and functional properties of dark chocolate. Velciov et al. (2021) reported on the proximate composition of dark chocolate containing 40%-80% cocoa mass.
Although the qualitative influence of fat content on chocolate rheology is well established in the literature (Glicerina et al., 2016; Vasquez et al., 2019), there is a paucity of comparative studies among commercially available dark chocolates to evaluate their rheological characteristics on cocoa mass content. These flow characteristics have a significant impact on product yields, production facility efficiency, transportation during the manufacturing process, and consumer preferences. Consequently, the evaluation of rheological properties is of paramount importance in practical applications concerning handling and quality control, as well as for engineering calculations. The objective of this study was to systematically investigate the rheological properties of dark chocolates, in order to establish a relationship between cocoa mass content and its temperature dependency.
Materials and Methods
Commercial dark chocolate samples containing 86% and 95% cocoa mass (Meiji Co., Ltd., Tokyo, Japan) and a cocoa mass sample (ECC NV, Malle, Belgium) were procured from a local market. The samples were designated as 86CM, 95CM, and 100CM, respectively, according to the concentration of cocoa mass in the sample. The samples were stored in a chiller at approximately 4°C and liquefied at 40°C prior to each analysis.
A 5 g sample was mixed with 45 mL of distilled water and homogenized for 1 min. The mixture was left at room temperature for 1 h to allow for separation of the solid and liquid phases. The pH of the resulting liquid was measured using a pH meter (pH/Ion 510, Oakton Instruments, Vernon Hills, IL, USA). The color determination of the samples on a petri dish was conducted using a Chromameter (CM-600d, Minolta Co., Osaka, Japan) set for CIELAB color space. Total soluble solids (TSS) were measured using a refractometer (PR-301, Atago Co., Tokyo, Japan). Moisture content was obtained by drying a specific amount (5 g) of the sample to a constant weight at 105°C in an oven (FOL-2, Jeio Tech Co., Ltd., Daejeon, Korea), and the results were reported on a wet basis. Five measurements were taken for each sample.
Steady shear rheological measurements were conducted using a rotational rheometer (RV1, Thermo Fisher Scientific Inc., Karlsruhe, Germany) at 25°C, 35°C, and 45°C. The sample temperature was controlled using a temperature-controlled recirculating water bath (RW-0525G, Jeio Tech Co., Ltd., Daejeon, Korea). Prior to the measurements, the rheometer was first zero-adjusted. The tube was filled with approximately 40 mL of the sample. The rotor and sample tube were attached to the rheometer for experimentation. Twelve shear rates, ranging from 1 to 50 s–1 were selected, and the measuring time was 120 s. The experiments were replicated three times, and the mean values were compared.
The shear rate rheological properties of chocolate samples can be described by the Herschel-Bulkley model, which is commonly used to explain the flow behavior of various food products (Alsalman & Ramaswamy, 2021; Ramirez-Brewer et al., 2023). This model can be used to demonstrate the shear rate dependency of the properties.
where, σ is the shear stress (Pa), σy is the yield stress (Pa), K is the consistency coefficient (Pa · sn), γ̇ is the shear rate (s–1), and n is the flow behavior index (dimensionless).
The shear stress and shear rate data for chocolates at different temperatures were fitted with various linear regression equations using the RheoWin Job Manager software. The regression equations with the highest coefficient of determination were selected. The adequacy of the derived Herschel-Bulkley equations in predicting the shear stress values at different temperatures was further evaluated using the mean relative percentage error (MRPE), as outlined in Quispe-Chambilla et al. (2022).
where, N is the number of experiments, σexp is the measured σ from experiments and σpre is the calculated σ.
The viscosity parameter of samples can be related to temperature using an Arrhenius-type equation, as demonstrated by Diamante & Liu (2016) and Choi & Lee (2017).
where, K is the consistency coefficient (Pa · sn), K0 is the pre-exponential constant (Pa · sn), Ea is the activation energy (J/mol), R is the universal gas constant (8.314 J/mol · K), and T is the absolute temperature (K).
The mean and standard deviation were used to express the collected data (n=5). The results were analyzed using one-way analysis of variance (ANOVA) with general linear models (GLM) in Statistical Analysis System ver. 9.4 software (SAS/STAT, Cary, NC, USA). Duncan’s multiple range test was used to compare means, and significance was defined at the 5% level.
Results and Discussion
Table 1 presents the key characteristics of the chocolate samples used in this study. The pH values of the samples ranged from 6.14 to 6.47 and were influenced by the cocoa mass content (p<0.05). The pH of 100CM was relatively low compared to other chocolates due to the absence of added sugar and the presence of only cocoa solids. Lee (2023) reported similar pH values ranging from 6.10 to 6.55, depending on the addition of wheat sprout powder. The chocolate samples’ soluble solids content ranged from 0.64 to 1.34 and was significantly affected by the amount of cocoa mass (p<0.05). The soluble solids content of the 86CM samples was found to be higher than that of the 100CM samples, which can be attributed to the additional sugars and other ingredients present in the formulation. The moisture content of the samples ranged from 1.29 to 1.66%, which is comparable to the range of 1.22–1.39% reported for dark chocolates by Velciov et al. (2021).
The L* coordinate values of the various chocolate samples exhibited a range of 16.71 to 30.37. The lightness values were found to be significantly higher in the 100CM samples that did not contain any added ingredients, such as sugar, soy lecithin, and sucrose fatty acids, in the formulation (p<0.05). Although no significant differences were found between the 86CM and 95CM samples (p>0.05), the 100CM samples were darker. The values for coordinates a* and b* ranged from 2.43 to 6.34 and 1.17 to 10.72, respectively, and showed a pattern similar to that of the lightness values. Puchol-Miquel et al. (2021) reported a similar range (13.2–14.7) in the lightness values of chocolates made from reconstituted cocoa liquor and high cocoa content.
Fig. 1 displays the shear stress and shear rate behavior of 86CM, 95CM, and 100CM samples at temperatures of 25°C, 35°C, and 45°C. The results demonstrate that the shear stress increases rapidly with increasing shear rate at the studied temperatures, indicating shear-thinning behavior due to shear stress. Previous studies on chocolate samples have also demonstrated non-Newtonian fluid behavior of the pseudoplastic type (Vasquez et al., 2019; Quispe-Chambilla et al., 2022).
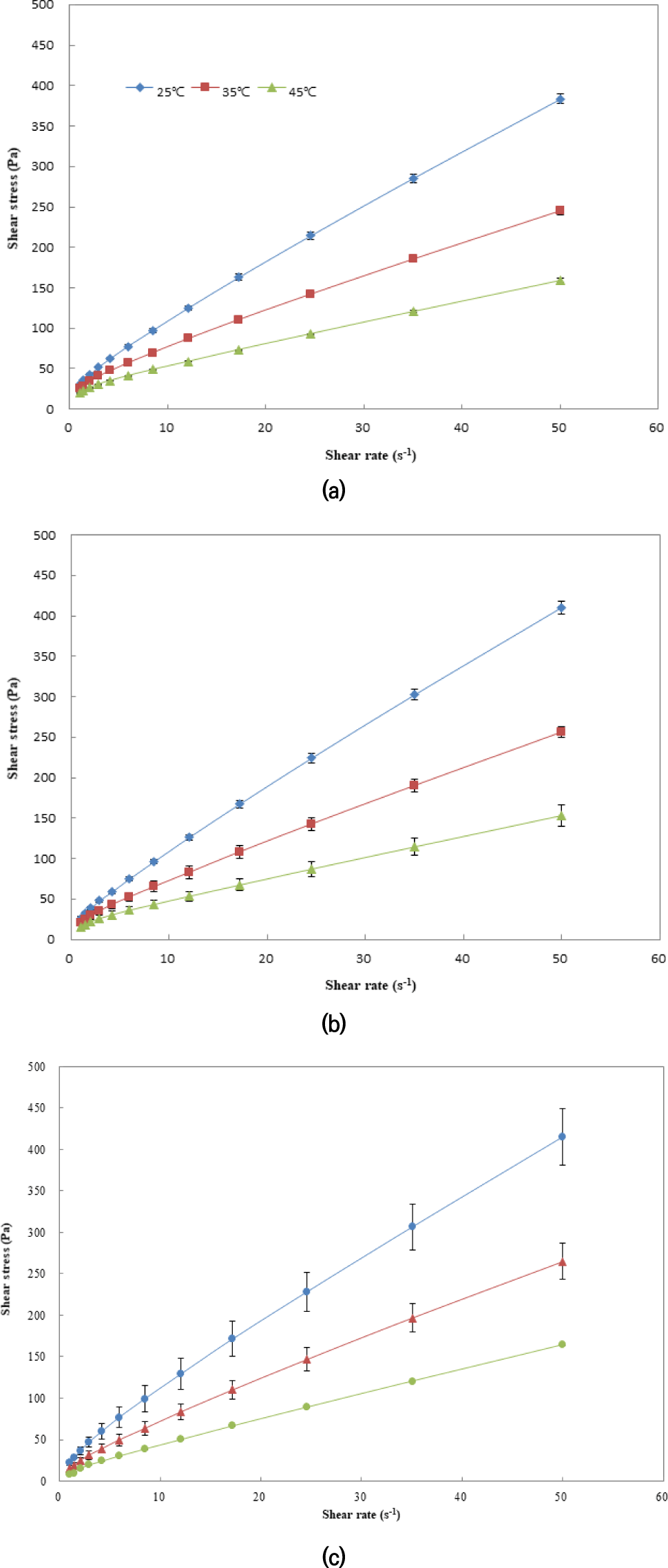
Table 2 presents the model constants obtained by fitting the Herschel-Bulkley model to experimental viscosity values as a function of shear rate within a shear rate range of 1–50 s–1 and the considered temperature range. The mean relative percentage error (MRPE) was calculated for experimental and predicted shear stresses in order to evaluate the equation’s goodness of fit. The MRPE values for the 86CM, 95CM, and 100CM samples were found to be in the range of 1.25–2.89, 0.87–3.45, and 2.21–4.97, respectively. All MRPE values were less than 10%, which is considered acceptable and suitable for most engineering purposes (Diamante & Liu, 2016; Pardeshi et al., 2009). The fitted model was confirmed to be adequate as all R2 values were higher than 0.99. All samples exhibited an expected pseudoplastic behavior with an initial resistance to flow (yield stress) and plastic viscosity. The mean n values were found to range between 0.83 and 0.89, which is consistent with the characteristics of a non-Newtonian fluid (Glicerina et al., 2016).
The yield stress values were observed to be significantly lower in 100CM samples compared to 86CM and 95CM samples at the same temperature (p<0.05). However, there was no significant difference was found between the 86CM and 95CM samples at the same temperature (p>0.05). Nevertheless, the yield stress values exhibited a tendency to decrease as the concentration of cocoa mass increased in the chocolate samples. This indicates that the samples with a higher concentration of cocoa mass require less energy to initiate fluid flow. This is consistent with the findings of Vasquez et al. (2019), where sample A (73% cocoa) underwent a solid-liquid transition at approximately 10 Pa, while sample D (41% cocoa) did so at approximately 500 Pa. This suggests that the stress required to overcome the initial interaction forces between fat and solid particles in chocolate matrices increases with decreasing fat content (Fernandes et al., 2013).
The consistency coefficient (K) is also used to characterize the flow properties of chocolate. A higher K value indicates a thicker, more viscous consistency. There were no significant differences in K values among samples at the same temperature (p>0.05), but decreased with increasing temperature within the sample. Changes in plastic viscosity are closely linked to the surface area of particles in contact with cocoa butter (Mongia & Ziegler, 2000) and the interaction between cocoa particles and cocoa fat (Do et al., 2007). Further research is required to clarify the effect of cocoa mass on K in these commercial samples, particularly in terms of particle size and distribution, which can significantly affect the rheological properties of chocolate samples.
The effect of temperature on chocolate rheology during steady shear was examined using an Arrhenius-type relationship. The pre-exponential constant (K0) and the activation energy (Ea) were determined from the slope and intercept of the Arrhenius regression, showing a good fit with R2>0.98 (Table 2). As previously stated, K decreases as temperature increases, in accordance with the typical trend of viscosity decreasing with temperature. The study found that the 100CM samples, which had the highest cocoa level, were the most temperature-sensitive. These samples exhibited a larger decrease in viscosity with increasing temperature due to their high activation energy of 39.64 kJ/mol. The 95CM samples had a slightly higher activation energy of 36.38 kJ/mol compared to the 86CM samples (32.54 kJ/mol). In a similar vein, Quispe-Chambilla et al. (2022) observed that dark chocolates with partial substitution of peanuts and Sacha Inchi exhibited activation energies in a comparable order of magnitude. Furthermore, Vasquez et al. (2019) also reported that the activation energy decreased with decreasing cocoa content of chocolate samples.
Conclusion
The study confirmed that dark commercial chocolates with varying amounts of cocoa mass at different temperatures (25°C, 35°C, and 45°C) exhibited non-Newtonian fluid behavior, as predicted by the Herschel-Bulkley model. The model was successfully used to construct the flow curves for the molten chocolate samples. The rheology of chocolate is significantly influenced by both cocoa mass content and temperature. The yield stress values decreased as the concentration of cocoa mass increased at the same temperature, and also with increasing temperature within the sample. No significant differences were found in the consistency coefficient due to cocoa mass content (p>0.05), but the consistency coefficient decreased with increasing temperature. The flow behavior index was not affected by any processing parameters, such as cocoa mass content and temperature (p>0.05). An Arrhenius-type relationship was used to relate the consistency coefficient temperature. The samples with the highest cocoa mass content exhibited the greatest temperature sensitivity, with an activation energy of 39.64 kJ/mol. The activation energy decreased as the cocoa content of the chocolate samples decreased.